![]() ![]() ![]() ![]() |
|||||||||||
![]() |
|||||||||||
|
![]() |
A 'butterfly'
(so-called because of its appearance) can be used to ensure that
an injection of radioactive material is inserted into the correct
site. It allows the doctor or nurse to check that the needle is
inserted properly before the radioactive fluid is pumped into
the patient. The fluid needs to be injected into the blood stream. If it goes into tissue it will be concentrated in a small area instead of being distributed throughout the blood (a large volume!) - it could therefore cause tissue damage. |
The radionuclide in the syringe is shielded to protect the person giving the injection. It is important to minimise the dose of personnel working with radioactive materials as each time they are exposed to radiation they increase the odds on their contracting cancer at a later date. |
![]() |
(Above
two images from CALRAD an interactive educational package designed by
an educational consortium of several Universities - I came across it
via the Open University module S803)
The gamma ray is electromagnetic radiation of very high penetration power. Therefore more rays exit the body and are available for detection than interact with the patient's tissue. The patient is now emitting gamma rays!
The doctor then gets the patient to wait for a while. This will give the tracer-chemical a chance to accumulate in the parts of the body the non-radioactive chemical would have done normally.
Cancerous
cells divide more frequently than non-cancerous ones and therefore a
'hot-spot' of high activity results
from any cancerous growth. A gamma camera is scheduled to scan
the area of interest approximately four hours after the patient has
been injected with the radioactive tracer. This gives it time to circulate
and accumulate in 'hot-spots' of rapid cell division. A second scan
is then taken within the 24-hour period and compared to the first to
diagnose suspicion of overactive lymph node activity.
- bone imaging,
- monitoring tumour metabolism,
- monitoring the function of the heart : cardiac perfusion and myocardial blood-flow monitoring,
- studying fatty acid metabolism
When a positron
is emitted it is soon annihilated and a pair of gamma
rays is produced.
These gamma rays fly off in opposite directions and can be viewed using a PET Scanner. This is a special type of gamma camera that is designed to count only gamma rays that are produced in pairs. It is linked to a computer that can deduce where the annihilation of the positron took place.
: Useful URLs :
There are many PET sites on the WWW but care has to be taken when searching because of confusion with 'pet animals'! (inclusion of the word 'gamma' usually overcomes this)
http://www.imaginis.com/nuclear-medicine/
http://www.nucmednet.com/frameset.htm
(this site has a 'real-time' image of a beating heart being monitored by gamma radiation)
A patient is injected with a radioactive tracer that emits gamma rays. The gamma ray is electromagnetic radiation of very high penetration power. Therefore more rays exit the body and are available for detection than interact with the patient's tissue. These can be detected by a gamma camera and the concentration of radioactive tracer in various parts of the body can be ascertained.
Gamma rays cannot be focused by refraction (like light rays can be) therefore a lead collimator is used to direct rays from a point on the patient towards a single point on a sodium iodide crystal. The collimator absorbs g-rays emanating from other parts of the body before they activate the crystal ensuring that only rays from the relevant part of the body hit the crystal
A crystal of sodium iodide fluoresces when a gamma ray interacts with one of its orbital electrons, promoting it to a higher energy level. On its return to ground state a photon is emitted. If this energy is in the visible region a flash of light is seen.
This light is detected
using a photomultiplier and changed
into an electrical output so that it can be displayed on a monitor.The
electrical output can be translated into colour coded graphical display
using electronic circuitry and the camera display can be made on a monitor
and videoed. Hard copies of the scans can then be taken and compared.
The electrical output can be translated into colour coded graphical display
using electronic circuitry.
![]() |
![]() |
![]()
|
|
Images taken
from: |
|
Above image taken from: http://www.sielimaging.com/images/gamma_cam1.jpg
Tumours
can be treated using gamma or X-rays.
Cells that divide rapidly are more prone to damage by high-energy electromagnetic
radiation. This means that tumour cells are more radiosensitive
than their normal counterparts. By carefully aiming the rays at the tumour
(gamma-ray beams directed from a multitude of angles that result in the
maximum gamma ray intensity within the tumour) the harmful effect of the
ionising radiation is kept to a minimum
in the surrounding tissue. This kind of treatment is most hazardous when
a brain tumour is being irradiated and the surrounding tissue is vital
for normal brain function. Several treatments are usually given over a
time period of several weeks to minimise the unpleasant side effects (most
commonly nausea, sickness, and tiredness).
New methods of delivering
radiation treatment have been developed
-
- Interstitial radiation involves implanting radioactive chemicals (termed seeds) directly into a tumour.
- Stereotactic radiosurgery delivers a high, single dose of radiation to a small, well-defined area.
http://www.times-archive.co.uk/JohnDiamond/ (An excellent resource for bringing home the 'human side' of treatments - select the article published 22nd July 2000 - he describes what its like on the receiving end of treatment requiring radioactive wires inserted into the neck)
http://www.salu.net/sites/r/rtsideffects/
(general information on possible side effects of treatment)
http://www.radiotherapy.com/education/index.htm
http://www.oncolink.com/specialty/rad_onc/general/xrt_intro.html
http://www.oncolink.com/pdq_html/6/engl/600071.html
http://www.biomed.org/pet.html#what
http://www.valley-radiotherapy.com/treatment/specific/index.asp
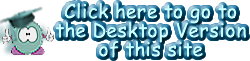